mirror of
https://github.com/bitcoinbook/bitcoinbook
synced 2025-04-18 08:09:08 +00:00
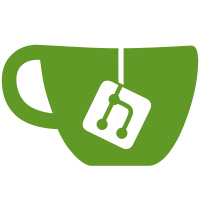
- Rename to HD Key Generation to avoid confusing use of the term "wallet" - Remove detail that's now redundant thanks to the introduction of the newly added previous sections. - Lightly edit the rest.
1002 lines
46 KiB
Plaintext
1002 lines
46 KiB
Plaintext
[[ch05_wallets]]
|
|
== Wallet Recovery
|
|
|
|
Creating pairs of private and public keys is a crucial part of allowing
|
|
Bitcoin wallets to receive and spend bitcoins. But losing access to a
|
|
private key can make it impossible for anyone to ever spend the bitcoins
|
|
received to the corresponding public key. Wallet and protocol
|
|
developers over the years have worked to design systems that allow users
|
|
to recover access to their bitcoins after a problem without compromising
|
|
security the rest of the time.
|
|
|
|
In this chapter, we'll examine some of the different methods employed by
|
|
wallets to prevent the loss of data from becoming a loss of money.
|
|
Some solutions have almost no downsides and are universally adopted by
|
|
modern wallets. We'll simply recommend those solutions as best
|
|
practices. Other solutions have both advantages and disadvantages,
|
|
leading different wallet authors to make different tradeoffs.
|
|
In those cases, we'll describe the various options available.
|
|
|
|
=== Independent Key Generation
|
|
|
|
((("wallets", "contents of")))Wallets for physical cash hold that cash,
|
|
so it's unsurprising that many people mistakenly believe that
|
|
bitcoin wallets contain bitcoins. In fact, what many people call a
|
|
Bitcoin wallet--which we call a _wallet database_ to distinguish it
|
|
from wallet applications--contains only keys. Those keys are associated
|
|
with bitcoins recorded on the blockchain. By proving to Bitcoin full nodes that you
|
|
control the keys, you can can spend the associated bitcoins.
|
|
|
|
Simple wallet databases contain both the public keys to which bitcoins
|
|
are received and the private keys which allow creating the signatures
|
|
necessary to authorize spending those bitcoins. Other wallets databases
|
|
may contain only public keys, or only some of the private keys necessary
|
|
to authorize a spending transaction. Their wallet applications produce
|
|
the necessary signatures by working with external tools, such as
|
|
hardware signing devices or other wallets in a multi-signature scheme.
|
|
|
|
It's possible for a wallet application to independently generate each of
|
|
the wallet keys it later plans to use. All early Bitcoin wallet applications did
|
|
this, but this required users back up the wallet database each time they
|
|
generated and distributed new keys, which could be as often as each time
|
|
they generated a new address to receive a new payment. Failure to back
|
|
up the wallet database on time would lead to the user losing access to
|
|
any funds received to keys which had not been backed up.
|
|
|
|
For each independently-generated key, the user would need to back up
|
|
about 32 bytes, plus overhead. Some users and wallet applications tried
|
|
to minimize the amount of data that needed to be backed up
|
|
by only using a single key. Although that can be secure, it severely
|
|
reduces the privacy of that user and all of the people with whom they
|
|
transact. People who valued their privacy and those of their peers
|
|
created new keypairs for each transaction, producing wallet databases
|
|
that could only reasonably be backed up using digital media.
|
|
|
|
[[Type0_wallet]]
|
|
[role="smallersixty"]
|
|
.Non-deterministic key generation: a collection of independently generated keys stored in a wallet database
|
|
image::images/mbc2_0501.png["Non-Deterministic Wallet"]
|
|
|
|
Modern wallet applications don't independently generate keys but instead
|
|
derive them from a single random seed using a repeatable (deterministic)
|
|
algorithm.
|
|
|
|
==== Deterministic Key Generation
|
|
|
|
A hash function will always produce the same output when given the same
|
|
input, but if the input is changed even slightly, the output will be
|
|
different. If the function is cryptographically secure, nobody should
|
|
be able to predict the new output--not even if they know the new input.
|
|
|
|
This allows us to take one random value and transform it into a
|
|
practically unlimited number of seemingly-random values. Even more
|
|
usefully, later using the same hash function with the same input
|
|
(called a _seed_) will produce the same seemingly-random values.
|
|
|
|
----
|
|
# Collect some entropy (randomness)
|
|
$ dd if=/dev/random count=1 status=none | sha256sum
|
|
f1cc3bc03ef51cb43ee7844460fa5049e779e7425a6349c8e89dfbb0fd97bb73 -
|
|
|
|
# Set our seed to the random value
|
|
$ seed=f1cc3bc03ef51cb43ee7844460fa5049e779e7425a6349c8e89dfbb0fd97bb73
|
|
|
|
# Deterministically generate derived values
|
|
$ for i in {0..2} ; do echo "$seed + $i" | sha256sum ; done
|
|
50b18e0bd9508310b8f699bad425efdf67d668cb2462b909fdb6b9bd2437beb3 -
|
|
a965dbcd901a9e3d66af11759e64a58d0ed5c6863e901dfda43adcd5f8c744f3 -
|
|
19580c97eb9048599f069472744e51ab2213f687d4720b0efc5bb344d624c3aa -
|
|
----
|
|
|
|
If we use the derived values as our private keys, we can later generate
|
|
exactly those same private keys by using our seed value with the
|
|
algorithm we used before. A user of deterministic key generation can
|
|
back up every key in their wallet by simply recording their seed and
|
|
a reference to the deterministic algorithm they used. For example, even
|
|
if Alice has a million bitcoins received to a million different
|
|
addresses, all she needs to back up in order to later recover access to
|
|
those bitcoins is:
|
|
|
|
----
|
|
f1cc 3bc0 3ef5 1cb4 3ee7 8444 60fa 5049
|
|
e779 e742 5a63 49c8 e89d fbb0 fd97 bb73
|
|
----
|
|
|
|
A logical diagram of basic sequential deterministic key generation is
|
|
shown in <<Type1_wallet>>. However, modern wallet applications have a
|
|
more clever way of accomplishing this that allows public keys to be
|
|
derived separately from their corresponding private keys, making it
|
|
possible to store private keys more securely than public keys.
|
|
|
|
[[Type1_wallet]]
|
|
[role="smallersixty"]
|
|
.Deterministic key generation: a deterministic sequence of keys derived from a seed for a wallet database
|
|
image::images/mbc2_0502.png["Deterministic Wallet"]
|
|
|
|
==== Public Child Key Derivation
|
|
|
|
In <<public_key_derivation>>, we learned how to create a public key from a private key
|
|
using Elliptic Curve Cryptography (ECC). Although operations on an
|
|
elliptic curve are not intuitive, they are analogous to the addition,
|
|
subtraction, and multiplication operations we use in regular
|
|
arithmetic. In other words, it's possible to add or subtract from a
|
|
public key, or to multiply it. Consider the equation we used for
|
|
generating a public key (K) from a private key (k) using the generator
|
|
point (G):
|
|
|
|
----
|
|
K == k * G
|
|
----
|
|
|
|
It's possible to create a derived keypair, called a child keypair, by
|
|
simply adding the same value to both sides of the equation:
|
|
|
|
----
|
|
K + (123 * G) == (k + 123) * G
|
|
----
|
|
|
|
An interesting consequence of this is that adding `123` to the public
|
|
key can be done using entirely public information. For example, Alice
|
|
generates public key K and gives it to Bob. Bob doesn't know the
|
|
private key, but he does know the global constant G, so he can add any
|
|
value to the public key to produce a derived public child key. If he
|
|
then tells Alice the value he added to the public key, she can add the
|
|
same value to the private key, producing a derived private child key
|
|
that corresponds to the public child key Bob created.
|
|
|
|
In other words, it's possible to create child public keys even if you
|
|
don't know anything about the parent private key. The value added to a
|
|
public key is known as a _key tweak._ If a deterministic algorithm is
|
|
used for generating the key tweaks, then it's possible to for someone
|
|
who doesn't know the private key to create an essentially unlimited
|
|
sequence of public child keys from a single public parent key. The
|
|
person who controls the private parent key can then use the same key
|
|
tweaks to create all the corresponding private child keys.
|
|
|
|
This technique is commonly used is to separate wallet application
|
|
frontends (which don't require private keys) from signing operations
|
|
(which do require private keys). For example, Alice's frontend
|
|
distributes her public keys to people wanting to pay her. Later, when
|
|
she wants to spend the received money, she can provide the key tweaks
|
|
she used to a _hardware signing device_ (sometimes confusingly called a
|
|
_hardware wallet_) which securely stores her original private key. The
|
|
hardware signer uses the tweaks to derive the necessary child private
|
|
keys and uses them to sign the transactions, returning the signed
|
|
transactions to the less-secure frontend for broadcast to the Bitcoin
|
|
network.
|
|
|
|
Public child key derivation can produce a linear sequence of keys
|
|
similar to the previously seen <<Type1_wallet>>, but modern wallets
|
|
applications use one more trick to provide a tree of keys instead a
|
|
single sequence.
|
|
|
|
[[hd_wallets]]
|
|
==== Hierarchical Deterministic (HD) Key Generation (BIP32)
|
|
|
|
Every modern Bitcoin wallet of which we're aware uses Hierarchical
|
|
Deterministic (HD) key generation by default. This standard, defined in
|
|
BIP32, uses deterministic key generation and optional public child key
|
|
derivation with an algorithm that produces a tree of keys.
|
|
In this tree, any key can be the parent of a sequence of child keys, and
|
|
any of those child keys can be a parent for another sequence of
|
|
child keys (grandchildren of the original key). There's no arbitrary
|
|
limit on the depth of the tree. This tree structure is illustrated in
|
|
<<Type2_wallet>>.
|
|
|
|
[[Type2_wallet]]
|
|
.HD wallet: a tree of keys generated from a single seed
|
|
image::images/mbc2_0503.png["HD wallet"]
|
|
|
|
The tree structure can be used to express additional
|
|
organizational meaning, such as when a specific branch of subkeys is
|
|
used to receive incoming payments and a different branch is used to
|
|
receive change from outgoing payments. Branches of keys can also be used
|
|
in corporate settings, allocating different branches to departments,
|
|
subsidiaries, specific functions, or accounting categories.
|
|
|
|
We'll provide a detailed exploration of HD wallets in <<hd_wallet_details>>.
|
|
|
|
==== Seeds and Mnemonic Codes (BIP39)
|
|
|
|
((("wallets", "technology of", "seeds and mnemonic codes")))((("mnemonic
|
|
code words")))((("bitcoin improvement proposals", "Mnemonic Code Words
|
|
(BIP39)")))HD wallets are a very powerful mechanism for managing many
|
|
keys and addresses. They are even more useful if they are combined with
|
|
a standardized way of creating seeds from a sequence of English words
|
|
that are easy to transcribe, export, and import across wallets. This is
|
|
known as a _mnemonic_ and the standard is defined by BIP39. Today, most
|
|
bitcoin wallets (as well as wallets for other cryptocurrencies) use this
|
|
standard and can import and export seeds for backup and recovery using
|
|
interoperable mnemonics.
|
|
|
|
Let's look at this from a practical perspective. Which of the following
|
|
seeds is easier to transcribe, record on paper, read without error,
|
|
export, and import into another wallet?
|
|
|
|
.A seed for an deterministic wallet, in hex
|
|
----
|
|
0C1E24E5917779D297E14D45F14E1A1A
|
|
----
|
|
|
|
.A seed for an deterministic wallet, from a 12-word mnemonic
|
|
----
|
|
army van defense carry jealous true
|
|
garbage claim echo media make crunch
|
|
----
|
|
|
|
==== Wallet Best Practices
|
|
|
|
((("wallets", "best practices for")))((("bitcoin improvement proposals",
|
|
"Multipurpose HD Wallet Structure (BIP43)")))As bitcoin wallet
|
|
technology has matured, certain common industry standards have emerged
|
|
that make bitcoin wallets broadly interoperable, easy to use, secure,
|
|
and flexible. These common standards are:
|
|
|
|
* Mnemonic code words, based on BIP39
|
|
* HD wallets, based on BIP32
|
|
* Multipurpose HD wallet structure, based on BIP43
|
|
* Multicurrency and multiaccount wallets, based on BIP44
|
|
|
|
These standards may change or may become obsolete by future
|
|
developments, but for now they form a set of interlocking technologies
|
|
that have become the de facto wallet standard for bitcoin.
|
|
|
|
The standards have been adopted by a broad range of software and
|
|
hardware bitcoin wallets, making all these wallets interoperable. A user
|
|
can export a mnemonic generated on one of these wallets and import it in
|
|
another wallet, recovering all transactions, keys, and addresses.
|
|
|
|
((("hardware wallets")))((("hardware wallets", see="also wallets")))Some
|
|
example of software wallets supporting these standards include (listed
|
|
alphabetically) Breadwallet, Copay, Multibit HD, and Mycelium. Examples
|
|
of hardware wallets supporting these standards include (listed
|
|
alphabetically) Keepkey, Ledger, and Trezor.
|
|
|
|
The following sections examine each of these technologies in detail.
|
|
|
|
[TIP]
|
|
====
|
|
If you are implementing a bitcoin wallet, it should be built as a HD
|
|
wallet, with a seed encoded as mnemonic code for backup, following the
|
|
BIP32, BIP39, BIP43, and BIP44 standards, as described in the
|
|
following sections.
|
|
====
|
|
|
|
==== Using a Bitcoin Wallet
|
|
|
|
((("wallets", "using bitcoin wallets")))In <<user-stories>> we
|
|
introduced Gabriel, ((("use cases", "web store", id="gabrielfive")))an
|
|
enterprising young teenager in Rio de Janeiro, who is running a simple
|
|
web store that sells bitcoin-branded t-shirts, coffee mugs, and
|
|
stickers.
|
|
|
|
((("wallets", "types of", "hardware wallets")))Gabriel uses a Trezor
|
|
bitcoin hardware wallet (<<a_trezor_device>>) to securely manage his
|
|
bitcoin. The Trezor is a simple USB device with two buttons that stores
|
|
keys (in the form of an HD wallet) and signs transactions. Trezor
|
|
wallets implement all the industry standards discussed in this chapter,
|
|
so Gabriel is not reliant on any proprietary technology or single vendor
|
|
solution.
|
|
|
|
[[a_trezor_device]]
|
|
.A Trezor device: a bitcoin HD wallet in hardware
|
|
image::images/mbc2_0504.png[alt]
|
|
|
|
When Gabriel used the Trezor for the first time, the device generated a
|
|
mnemonic and seed from a built-in hardware random number generator.
|
|
During this initialization phase, the wallet displayed a numbered
|
|
sequence of words, one by one, on the screen (see
|
|
<<trezor_mnemonic_display>>).
|
|
|
|
[[trezor_mnemonic_display]]
|
|
.Trezor displaying one of the mnemonic words
|
|
image::images/mbc2_0505.png["Trezor wallet display of mnemonic word"]
|
|
|
|
By writing down this mnemonic, Gabriel created a backup (see
|
|
<<mnemonic_paper_backup>>) that can be used for recovery in the case of
|
|
loss or damage to the Trezor device. This mnemonic can be used for
|
|
recovery in a new Trezor or in any one of the many compatible software
|
|
or hardware wallets. Note that the sequence of words is important, so
|
|
mnemonic paper backups have numbered spaces for each word. Gabriel had
|
|
to carefully record each word in the numbered space to preserve the
|
|
correct sequence.
|
|
|
|
[[mnemonic_paper_backup]]
|
|
.Gabriel's paper backup of the mnemonic
|
|
[cols="<1,^50,<1,^50", width="80%"]
|
|
|===
|
|
|*1.*| _army_ |*7.*| _garbage_
|
|
|*2.*| _van_ |*8.*| _claim_
|
|
|*3.*| _defense_ |*9.*| _echo_
|
|
|*4.*| _carry_ |*10.*| _media_
|
|
|*5.*| _jealous_ |*11.*| _make_
|
|
|*6.*| _true_ |*12.*| _crunch_
|
|
|===
|
|
|
|
[NOTE]
|
|
====
|
|
A 12-word mnemonic is shown in <<mnemonic_paper_backup>>, for
|
|
simplicity. In fact, most hardware wallets generate a more secure
|
|
24-word mnemonic. The mnemonic is used in exactly the same way,
|
|
regardless of length.
|
|
====
|
|
|
|
For the first implementation of his web store, Gabriel uses a single
|
|
Bitcoin address, generated on his Trezor device. This single address is
|
|
used by all customers for all orders. As we will see, this approach has
|
|
some drawbacks and can be improved upon with an HD wallet.((("",
|
|
startref="gabrielfive")))
|
|
|
|
=== Wallet Technology Details
|
|
|
|
Let's now examine each of the important industry standards that are used
|
|
by many bitcoin wallets in detail.
|
|
|
|
[[mnemonic_code_words]]
|
|
==== Mnemonic Code Words (BIP39)
|
|
|
|
((("wallets", "technology of", "mnemonic code words")))((("mnemonic code
|
|
words", id="mnemonic05")))((("bitcoin improvement proposals", "Mnemonic
|
|
Code Words (BIP39)", id="BIP3905")))Mnemonic code words are word
|
|
sequences that represent (encode) a random number used as a seed to
|
|
derive a deterministic wallet. The sequence of words is sufficient to
|
|
re-create the seed and from there re-create the wallet and all the
|
|
derived keys. A wallet application that implements deterministic wallets
|
|
with mnemonic words will show the user a sequence of 12 to 24 words when
|
|
first creating a wallet. That sequence of words is the wallet backup and
|
|
can be used to recover and re-create all the keys in the same or any
|
|
compatible wallet application. Mnemonic words make it easier for users
|
|
to back up wallets because they are easy to read and correctly
|
|
transcribe, as compared to a random sequence of numbers.
|
|
|
|
[TIP]
|
|
====
|
|
((("brainwallets")))Mnemonic words are often confused with
|
|
"brainwallets." They are not the same. The primary difference is that a
|
|
brainwallet consists of words chosen by the user, whereas mnemonic words
|
|
are created randomly by the wallet and presented to the user. This
|
|
important difference makes mnemonic words much more secure, because
|
|
humans are very poor sources of randomness.
|
|
====
|
|
|
|
Mnemonic codes are defined in BIP39 (see <<appdxbitcoinimpproposals>>).
|
|
Note that BIP39 is one implementation of a mnemonic code standard.
|
|
((("Electrum wallet", seealso="wallets")))There is a different standard,
|
|
with a different set of words, used by the Electrum wallet and predating
|
|
BIP39. BIP39 was proposed by the company behind the Trezor hardware
|
|
wallet and is incompatible with Electrum's implementation. However,
|
|
BIP39 has now achieved broad industry support across dozens of
|
|
interoperable implementations and should be considered the de facto
|
|
industry standard.
|
|
|
|
BIP39 defines the creation of a mnemonic code and seed, which we
|
|
describe here in nine steps. For clarity, the process is split into two
|
|
parts: steps 1 through 6 are shown in <<generating_mnemonic_words>> and
|
|
steps 7 through 9 are shown in <<mnemonic_to_seed>>.
|
|
|
|
[[generating_mnemonic_words]]
|
|
===== Generating mnemonic words
|
|
|
|
Mnemonic words are generated automatically by the wallet using the
|
|
standardized process defined in BIP39. The wallet starts from a source
|
|
of entropy, adds a checksum, and then maps the entropy to a word list:
|
|
|
|
1. Create a random sequence (entropy) of 128 to 256 bits.
|
|
|
|
2. Create a checksum of the random sequence by taking the first
|
|
(entropy-length/32) bits of its SHA256 hash.
|
|
|
|
3. Add the checksum to the end of the random sequence.
|
|
|
|
4. Split the result into 11-bit length segments.
|
|
|
|
5. Map each 11-bit value to a word from the predefined dictionary of
|
|
2048 words.
|
|
|
|
6. The mnemonic code is the sequence of words.
|
|
|
|
<<generating_entropy_and_encoding>> shows how entropy is used to
|
|
generate mnemonic words.
|
|
|
|
[[generating_entropy_and_encoding]]
|
|
[role="smallerseventy"]
|
|
.Generating entropy and encoding as mnemonic words
|
|
image::images/mbc2_0506.png["Generating entropy and encoding as mnemonic words"]
|
|
|
|
<<table_4-5>> shows the relationship between the size of the entropy
|
|
data and the length of mnemonic codes in words.
|
|
|
|
[[table_4-5]]
|
|
.Mnemonic codes: entropy and word length
|
|
[options="header"]
|
|
|=======
|
|
|Entropy (bits) | Checksum (bits) | Entropy *+* checksum (bits) | Mnemonic length (words)
|
|
| 128 | 4 | 132 | 12
|
|
| 160 | 5 | 165 | 15
|
|
| 192 | 6 | 198 | 18
|
|
| 224 | 7 | 231 | 21
|
|
| 256 | 8 | 264 | 24
|
|
|=======
|
|
|
|
[[mnemonic_to_seed]]
|
|
===== From mnemonic to seed
|
|
|
|
((("key-stretching function")))((("PBKDF2 function")))The mnemonic words
|
|
represent entropy with a length of 128 to 256 bits. The entropy is then
|
|
used to derive a longer (512-bit) seed through the use of the
|
|
key-stretching function PBKDF2. The seed produced is then used to build
|
|
a deterministic wallet and derive its keys.
|
|
|
|
((("salts")))((("passphrases")))The key-stretching function takes two
|
|
parameters: the mnemonic and a _salt_. The purpose of a salt in a
|
|
key-stretching function is to make it difficult to build a lookup table
|
|
enabling a brute-force attack. In the BIP39 standard, the salt has
|
|
another purpose—it allows the introduction of a passphrase that
|
|
serves as an additional security factor protecting the seed, as we will
|
|
describe in more detail in <<mnemonic_passphrase>>.
|
|
|
|
The process described in steps 7 through 9 continues from the process
|
|
described previously in <<generating_mnemonic_words>>:
|
|
|
|
++++
|
|
<ol start="7">
|
|
<li>The first parameter to the PBKDF2 key-stretching function is the
|
|
<em>mnemonic</em> produced from step 6.</li>
|
|
|
|
<li>The second parameter to the PBKDF2 key-stretching function is a
|
|
<em>salt</em>. The salt is composed of the string constant
|
|
"<code>mnemonic</code>" concatenated with an optional user-supplied
|
|
passphrase string.</li>
|
|
|
|
<li>PBKDF2 stretches the mnemonic and salt parameters using 2048
|
|
rounds of hashing with the HMAC-SHA512 algorithm, producing a 512-bit
|
|
value as its final output. That 512-bit value is the seed.</li>
|
|
</ol>
|
|
++++
|
|
|
|
<<fig_5_7>> shows how a mnemonic is used to generate a seed.
|
|
|
|
[[fig_5_7]]
|
|
.From mnemonic to seed
|
|
image::images/mbc2_0507.png["From mnemonic to seed"]
|
|
|
|
[TIP]
|
|
====
|
|
The key-stretching function, with its 2048 rounds of hashing, is a very
|
|
effective protection against brute-force attacks against the mnemonic or
|
|
the passphrase. It makes it extremely costly (in computation) to try
|
|
more than a few thousand passphrase and mnemonic combinations, while the
|
|
number of possible derived seeds is vast (2^512^).
|
|
====
|
|
|
|
Tables pass:[<a data-type="xref" href="#mnemonic_128_no_pass"
|
|
data-xrefstyle="select: labelnumber">#mnemonic_128_no_pass</a>],
|
|
pass:[<a data-type="xref" href="#mnemonic_128_w_pass"
|
|
data-xrefstyle="select: labelnumber">#mnemonic_128_w_pass</a>], and
|
|
pass:[<a data-type="xref" href="#mnemonic_256_no_pass"
|
|
data-xrefstyle="select: labelnumber">#mnemonic_256_no_pass</a>] show
|
|
some examples of mnemonic codes and the seeds they produce (without any
|
|
passphrase).
|
|
|
|
[[mnemonic_128_no_pass]]
|
|
.128-bit entropy mnemonic code, no passphrase, resulting seed
|
|
[cols="h,"]
|
|
|=======
|
|
| *Entropy input (128 bits)*| +0c1e24e5917779d297e14d45f14e1a1a+
|
|
| *Mnemonic (12 words)* | +army van defense carry jealous true garbage claim echo media make crunch+
|
|
| *Passphrase*| (none)
|
|
| *Seed (512 bits)* | +5b56c417303faa3fcba7e57400e120a0ca83ec5a4fc9ffba757fbe63fbd77a89a1a3be4c67196f57c39+
|
|
+a88b76373733891bfaba16ed27a813ceed498804c0570+
|
|
|=======
|
|
|
|
[[mnemonic_128_w_pass]]
|
|
.128-bit entropy mnemonic code, with passphrase, resulting seed
|
|
[cols="h,"]
|
|
|=======
|
|
| *Entropy input (128 bits)*| +0c1e24e5917779d297e14d45f14e1a1a+
|
|
| *Mnemonic (12 words)* | +army van defense carry jealous true garbage claim echo media make crunch+
|
|
| *Passphrase*| SuperDuperSecret
|
|
| *Seed (512 bits)* | +3b5df16df2157104cfdd22830162a5e170c0161653e3afe6c88defeefb0818c793dbb28ab3ab091897d0+
|
|
+715861dc8a18358f80b79d49acf64142ae57037d1d54+
|
|
|=======
|
|
|
|
|
|
[[mnemonic_256_no_pass]]
|
|
.256-bit entropy mnemonic code, no passphrase, resulting seed
|
|
[cols="h,"]
|
|
|=======
|
|
| *Entropy input (256 bits)* | +2041546864449caff939d32d574753fe684d3c947c3346713dd8423e74abcf8c+
|
|
| *Mnemonic (24 words)* | +cake apple borrow silk endorse fitness top denial coil riot stay wolf
|
|
luggage oxygen faint major edit measure invite love trap field dilemma oblige+
|
|
| *Passphrase*| (none)
|
|
| *Seed (512 bits)* | +3269bce2674acbd188d4f120072b13b088a0ecf87c6e4cae41657a0bb78f5315b33b3a04356e53d062e5+
|
|
+5f1e0deaa082df8d487381379df848a6ad7e98798404+
|
|
|=======
|
|
|
|
[[mnemonic_passphrase]]
|
|
===== Optional passphrase in BIP39
|
|
|
|
((("passphrases")))The BIP39 standard allows the use of an optional
|
|
passphrase in the derivation of the seed. If no passphrase is used, the
|
|
mnemonic is stretched with a salt consisting of the constant string
|
|
+"mnemonic"+, producing a specific 512-bit seed from any given mnemonic.
|
|
If a passphrase is used, the stretching function produces a _different_
|
|
seed from that same mnemonic. In fact, given a single mnemonic, every
|
|
possible passphrase leads to a different seed. Essentially, there is no
|
|
"wrong" passphrase. All passphrases are valid and they all lead to
|
|
different seeds, forming a vast set of possible uninitialized wallets.
|
|
The set of possible wallets is so large (2^512^) that there is no
|
|
practical possibility of brute-forcing or accidentally guessing one that
|
|
is in use.
|
|
|
|
[TIP]
|
|
====
|
|
There are no "wrong" passphrases in BIP39. Every passphrase leads to
|
|
some wallet, which unless previously used will be empty.
|
|
====
|
|
|
|
The optional passphrase creates two important features:
|
|
|
|
- A second factor (something memorized) that makes a mnemonic useless on
|
|
its own, protecting mnemonic backups from compromise by a thief.
|
|
|
|
- A form of plausible deniability or "duress wallet," where a chosen
|
|
passphrase leads to a wallet with a small amount of funds used to
|
|
distract an attacker from the "real" wallet that contains the majority
|
|
of funds.
|
|
|
|
However, it is important to note that the use of a passphrase also introduces the risk of loss:
|
|
|
|
* If the wallet owner is incapacitated or dead and no one else knows the passphrase, the seed is useless and all the funds stored in the wallet are lost forever.
|
|
|
|
* Conversely, if the owner backs up the passphrase in the same place as the seed, it defeats the purpose of a second factor.
|
|
|
|
While passphrases are very useful, they should only be used in
|
|
combination with a carefully planned process for backup and recovery,
|
|
considering the possibility of surviving the owner and allowing his or
|
|
her family to recover the cryptocurrency estate.
|
|
|
|
===== Working with mnemonic codes
|
|
|
|
BIP39 is implemented as a library in many different programming
|
|
languages:
|
|
|
|
https://github.com/trezor/python-mnemonic[python-mnemonic]:: The
|
|
reference implementation of the standard by the SatoshiLabs team that
|
|
proposed BIP39, in Python
|
|
|
|
https://github.com/bitcoinjs/bip39[bitcoinjs/bip39]:: An implementation
|
|
of BIP39, as part of the popular bitcoinJS framework, in JavaScript
|
|
|
|
https://github.com/libbitcoin/libbitcoin/blob/master/src/wallet/mnemonic.cpp[libbitcoin/mnemonic]::
|
|
An implementation of BIP39, as part of the popular Libbitcoin
|
|
framework, in pass:[<span class="keep-together">C++</span>]
|
|
|
|
There is also a BIP39 generator implemented in a standalone webpage,
|
|
which is extremely useful for testing and experimentation.
|
|
<<a_bip39_generator_as_a_standalone_web_page>> shows a standalone web
|
|
page that generates mnemonics, seeds, and extended private keys.
|
|
|
|
[[a_bip39_generator_as_a_standalone_web_page]]
|
|
.A BIP39 generator as a standalone web page
|
|
image::images/mbc2_0508.png["BIP39 generator web-page"]
|
|
|
|
((("", startref="mnemonic05")))((("", startref="BIP3905")))The page
|
|
(https://iancoleman.github.io/bip39/) can be used offline in a browser,
|
|
or accessed online.
|
|
|
|
==== Creating an HD Wallet from the Seed
|
|
|
|
((("wallets", "technology of", "creating HD wallets from root
|
|
seed")))((("root seeds")))((("hierarchical deterministic (HD)
|
|
wallets")))HD wallets are created from a single _root seed_, which is a
|
|
128-, 256-, or 512-bit random number. Most commonly, this seed is
|
|
generated from a _mnemonic_ as detailed in the previous section.
|
|
|
|
Every key in the HD wallet is deterministically derived from this root
|
|
seed, which makes it possible to re-create the entire HD wallet from
|
|
that seed in any compatible HD wallet. This makes it easy to back up,
|
|
restore, export, and import HD wallets containing thousands or even
|
|
millions of keys by simply transferring only the mnemonic that the root
|
|
seed is derived from.
|
|
|
|
The process of creating the master keys and master chain code for an HD
|
|
wallet is shown in <<HDWalletFromSeed>>.
|
|
|
|
[[HDWalletFromSeed]]
|
|
.Creating master keys and chain code from a root seed
|
|
image::images/mbc2_0509.png["HDWalletFromRootSeed"]
|
|
|
|
The root seed is input into the HMAC-SHA512 algorithm and the resulting
|
|
hash is used to create a _master private key_ (m) and a _master chain
|
|
code_ (c).
|
|
|
|
The master private key (m) then generates a corresponding master public
|
|
key (M) using the normal elliptic curve multiplication process +m * G+
|
|
that we saw in <<pubkey>>.
|
|
|
|
The chain code (c) is used to introduce entropy in the function that
|
|
creates child keys from parent keys, as we will see in the next section.
|
|
|
|
===== Private child key derivation
|
|
|
|
((("child key derivation (CKD)")))((("public and private keys", "child
|
|
key derivation (CKD)")))HD wallets use a _child key derivation_ (CKD)
|
|
function to derive child keys from parent keys.
|
|
|
|
The child key derivation functions are based on a one-way hash function
|
|
that combines:
|
|
|
|
* A parent private or public key (ECDSA uncompressed key)
|
|
* A seed called a chain code (256 bits)
|
|
* An index number (32 bits)
|
|
|
|
The chain code is used to introduce deterministic random data to the
|
|
process, so that knowing the index and a child key is not sufficient to
|
|
derive other child keys. Knowing a child key does not make it possible
|
|
to find its siblings, unless you also have the chain code. The initial
|
|
chain code seed (at the root of the tree) is made from the seed, while
|
|
subsequent child chain codes are derived from each parent chain code.
|
|
|
|
These three items (parent key, chain code, and index) are combined and
|
|
hashed to generate children keys, as follows.
|
|
|
|
The parent public key, chain code, and the index number are combined and
|
|
hashed with the HMAC-SHA512 algorithm to produce a 512-bit hash. This
|
|
512-bit hash is split into two 256-bit halves. The right-half 256 bits
|
|
of the hash output become the chain code for the child. The left-half
|
|
256 bits of the hash are added to the parent private key to produce the
|
|
child private key. In <<CKDpriv>>, we see this illustrated with the
|
|
index set to 0 to produce the "zero" (first by index) child of the
|
|
parent.
|
|
|
|
[[CKDpriv]]
|
|
.Extending a parent private key to create a child private key
|
|
image::images/mbc2_0510.png["ChildPrivateDerivation"]
|
|
|
|
Changing the index allows us to extend the parent and create the other
|
|
children in the sequence, e.g., Child 0, Child 1, Child 2, etc. Each
|
|
parent key can have 2,147,483,647 (2^31^) children (2^31^ is half of the
|
|
entire 2^32^ range available because the other half is reserved for a
|
|
special type of derivation we will talk about later in this chapter).
|
|
|
|
Repeating the process one level down the tree, each child can in turn
|
|
become a parent and create its own children, in an infinite number of
|
|
generations.
|
|
|
|
===== Using derived child keys
|
|
|
|
Child private keys are indistinguishable from nondeterministic (random)
|
|
keys. Because the derivation function is a one-way function, the child
|
|
key cannot be used to find the parent key. The child key also cannot be
|
|
used to find any siblings. If you have the n~th~ child, you cannot find
|
|
its siblings, such as the n–1 child or the n+1 child, or any
|
|
other children that are part of the sequence. Only the parent key and
|
|
chain code can derive all the children. Without the child chain code,
|
|
the child key cannot be used to derive any grandchildren either. You
|
|
need both the child private key and the child chain code to start a new
|
|
branch and derive grandchildren.
|
|
|
|
So what can the child private key be used for on its own? It can be used
|
|
to make a public key and a Bitcoin address. Then, it can be used to sign
|
|
transactions to spend anything paid to that address.
|
|
|
|
[TIP]
|
|
====
|
|
A child private key, the corresponding public key, and the Bitcoin
|
|
address are all indistinguishable from keys and addresses created
|
|
randomly. The fact that they are part of a sequence is not visible
|
|
outside of the HD wallet function that created them. Once created, they
|
|
operate exactly as "normal" keys.
|
|
====
|
|
|
|
===== Extended keys
|
|
|
|
((("public and private keys", "extended keys")))((("extended keys")))As
|
|
we saw earlier, the key derivation function can be used to create
|
|
children at any level of the tree, based on the three inputs: a key, a
|
|
chain code, and the index of the desired child. The two essential
|
|
ingredients are the key and chain code, and combined these are called an
|
|
_extended key_. The term "extended key" could also be thought of as
|
|
"extensible key" because such a key can be used to derive children.
|
|
|
|
Extended keys are stored and represented simply as the concatenation of
|
|
the 256-bit key and 256-bit chain code into a 512-bit sequence. There
|
|
are two types of extended keys. An extended private key is the
|
|
combination of a private key and chain code and can be used to derive
|
|
child private keys (and from them, child public keys). An extended
|
|
public key is a public key and chain code, which can be used to create
|
|
child public keys (_public only_), as described in
|
|
<<public_key_derivation>>.
|
|
|
|
Think of an extended key as the root of a branch in the tree structure
|
|
of the HD wallet. With the root of the branch, you can derive the rest
|
|
of the branch. The extended private key can create a complete branch,
|
|
whereas the extended public key can _only_ create a branch of public
|
|
keys.
|
|
|
|
[TIP]
|
|
====
|
|
An extended key consists of a private or public key and chain code. An
|
|
extended key can create children, generating its own branch in the tree
|
|
structure. Sharing an extended key gives access to the entire branch.
|
|
====
|
|
|
|
Extended keys are encoded using Base58Check, to easily export and import
|
|
between different BIP32–compatible wallets. The Base58Check
|
|
coding for extended keys uses a special version number that results in
|
|
the prefix "xprv" and "xpub" when encoded in Base58 characters to make
|
|
them easily recognizable. Because the extended key is 512 or 513 bits,
|
|
it is also much longer than other Base58Check-encoded strings we have
|
|
seen previously.
|
|
|
|
Here's an example of an extended _private_ key, encoded in Base58Check:
|
|
|
|
----
|
|
xprv9tyUQV64JT5qs3RSTJkXCWKMyUgoQp7F3hA1xzG6ZGu6u6Q9VMNjGr67Lctvy5P8oyaYAL9CAWrUE9i6GoNMKUga5biW6Hx4tws2six3b9c
|
|
----
|
|
|
|
Here's the corresponding extended _public_ key, encoded in Base58Check:
|
|
|
|
----
|
|
xpub67xpozcx8pe95XVuZLHXZeG6XWXHpGq6Qv5cmNfi7cS5mtjJ2tgypeQbBs2UAR6KECeeMVKZBPLrtJunSDMstweyLXhRgPxdp14sk9tJPW9
|
|
----
|
|
|
|
[[public__child_key_derivation]]
|
|
===== Public child key derivation
|
|
|
|
((("public and private keys", "public child key derivation")))As
|
|
mentioned previously, a very useful characteristic of HD wallets is the
|
|
ability to derive public child keys from public parent keys, _without_
|
|
having the private keys. This gives us two ways to derive a child public
|
|
key: either from the child private key, or directly from the parent
|
|
public key.
|
|
|
|
An extended public key can be used, therefore, to derive all of the
|
|
_public_ keys (and only the public keys) in that branch of the HD wallet
|
|
structure.
|
|
|
|
This shortcut can be used to create very secure public key–only
|
|
deployments where a server or application has a copy of an extended
|
|
public key and no private keys whatsoever. That kind of deployment can
|
|
produce an infinite number of public keys and Bitcoin addresses, but
|
|
cannot spend any of the money sent to those addresses. Meanwhile, on
|
|
another, more secure server, the extended private key can derive all the
|
|
corresponding private keys to sign transactions and spend the money.
|
|
|
|
One common application of this solution is to install an extended public
|
|
key on a web server that serves an ecommerce application. The web server
|
|
can use the public key derivation function to create a new Bitcoin
|
|
address for every transaction (e.g., for a customer shopping cart). The
|
|
web server will not have any private keys that would be vulnerable to
|
|
theft. Without HD wallets, the only way to do this is to generate
|
|
thousands of Bitcoin addresses on a separate secure server and then
|
|
preload them on the ecommerce server. That approach is cumbersome and
|
|
requires constant maintenance to ensure that the ecommerce server
|
|
doesn't "run out" of keys.
|
|
|
|
((("cold storage")))((("storage", "cold storage")))((("hardware
|
|
wallets")))Another common application of this solution is for
|
|
cold-storage or hardware wallets. In that scenario, the extended private
|
|
key can be stored on a paper wallet or hardware device (such as a Trezor
|
|
hardware wallet), while the extended public key can be kept online. The
|
|
user can create "receive" addresses at will, while the private keys are
|
|
safely stored offline. To spend the funds, the user can use the extended
|
|
private key on an offline signing Bitcoin client or sign transactions on
|
|
the hardware wallet device (e.g., Trezor). <<CKDpub>> illustrates the
|
|
mechanism for extending a parent public key to derive child public keys.
|
|
|
|
[[CKDpub]]
|
|
.Extending a parent public key to create a child public key
|
|
image::images/mbc2_0511.png["ChildPublicDerivation"]
|
|
|
|
==== Using an Extended Public Key on a Web Store
|
|
|
|
((("wallets", "technology of", "using extended public keys on web
|
|
stores")))Let's see how HD wallets are used by continuing our story with
|
|
Gabriel's web store.((("use cases", "web store", id="gabrielfivetwo")))
|
|
|
|
Gabriel first set up his web store as a hobby, based on a simple hosted
|
|
Wordpress page. His store was quite basic with only a few pages and an
|
|
order form with a single bitcoin address.
|
|
|
|
Gabriel used the first bitcoin address generated by his Trezor device as
|
|
the main bitcoin address for his store. This way, all incoming payments
|
|
would be paid to an address controlled by his Trezor hardware wallet.
|
|
|
|
Customers would submit an order using the form and send payment to
|
|
Gabriel's published bitcoin address, triggering an email with the order
|
|
details for Gabriel to process. With just a few orders each week, this
|
|
system worked well enough.
|
|
|
|
However, the little web store became quite successful and attracted many
|
|
orders from the local community. Soon, Gabriel was overwhelmed. With all
|
|
the orders paying the same address, it became difficult to correctly
|
|
match orders and transactions, especially when multiple orders for the
|
|
same amount came in close together.
|
|
|
|
Gabriel's HD wallet offers a much better solution through the ability to
|
|
derive public child keys without knowing the private keys. Gabriel can
|
|
load an extended public key (xpub) on his website, which can be used to
|
|
derive a unique address for every customer order. Gabriel can spend the
|
|
funds from his Trezor, but the xpub loaded on the website can only
|
|
generate addresses and receive funds. This feature of HD wallets is a
|
|
great security feature. Gabriel's website does not contain any private
|
|
keys and therefore does not need high levels of security.
|
|
|
|
To export the xpub, Gabriel uses the web-based software in conjunction
|
|
with the Trezor hardware wallet. The Trezor device must be plugged in
|
|
for the public keys to be exported. Note that hardware wallets will
|
|
never export private keys—those always remain on the device.
|
|
<<export_xpub>> shows the web interface Gabriel uses to export the xpub.
|
|
|
|
[[export_xpub]]
|
|
.Exporting an xpub from a Trezor hardware wallet
|
|
image::images/mbc2_0512.png["Exporting the xpub from the Trezor"]
|
|
|
|
Gabriel copies the xpub to his web store's bitcoin shop software. He
|
|
uses _Mycelium Gear_, which is an open source web-store plugin for a
|
|
variety of web hosting and content platforms. Mycelium Gear uses the
|
|
xpub to generate a unique address for every purchase. ((("",
|
|
startref="gabrielfivetwo")))
|
|
|
|
===== Hardened child key derivation
|
|
|
|
((("public and private keys", "hardened child key
|
|
derivation")))((("hardened derivation")))The ability to derive a branch
|
|
of public keys from an xpub is very useful, but it comes with a
|
|
potential risk. Access to an xpub does not give access to child private
|
|
keys. However, because the xpub contains the chain code, if a child
|
|
private key is known, or somehow leaked, it can be used with the chain
|
|
code to derive all the other child private keys. A single leaked child
|
|
private key, together with a parent chain code, reveals all the private
|
|
keys of all the children. Worse, the child private key together with a
|
|
parent chain code can be used to deduce the parent private key.
|
|
|
|
To counter this risk, HD wallets use an alternative derivation function
|
|
called _hardened derivation_, which "breaks" the relationship between
|
|
parent public key and child chain code. The hardened derivation function
|
|
uses the parent private key to derive the child chain code, instead of
|
|
the parent public key. This creates a "firewall" in the parent/child
|
|
sequence, with a chain code that cannot be used to compromise a parent
|
|
or sibling private key. The hardened derivation function looks almost
|
|
identical to the normal child private key derivation, except that the
|
|
parent private key is used as input to the hash function, instead of the
|
|
parent public key, as shown in the diagram in <<CKDprime>>.
|
|
|
|
[[CKDprime]]
|
|
.Hardened derivation of a child key; omits the parent public key
|
|
image::images/mbc2_0513.png["ChildHardPrivateDerivation"]
|
|
|
|
[role="pagebreak-before"]
|
|
When the hardened private derivation function is used, the resulting
|
|
child private key and chain code are completely different from what
|
|
would result from the normal derivation function. The resulting "branch"
|
|
of keys can be used to produce extended public keys that are not
|
|
vulnerable, because the chain code they contain cannot be exploited to
|
|
reveal any private keys. Hardened derivation is therefore used to create
|
|
a "gap" in the tree above the level where extended public keys are used.
|
|
|
|
In simple terms, if you want to use the convenience of an xpub to derive
|
|
branches of public keys, without exposing yourself to the risk of a
|
|
leaked chain code, you should derive it from a hardened parent, rather
|
|
than a normal parent. As a best practice, the level-1 children of the
|
|
master keys are always derived through the hardened derivation, to
|
|
prevent compromise of the master keys.
|
|
|
|
===== Index numbers for normal and hardened derivation
|
|
|
|
The index number used in the derivation function is a 32-bit integer. To
|
|
easily distinguish between keys derived through the normal derivation
|
|
function versus keys derived through hardened derivation, this index
|
|
number is split into two ranges. Index numbers between 0 and
|
|
2^31^–1 (0x0 to 0x7FFFFFFF) are used _only_ for normal
|
|
derivation. Index numbers between 2^31^ and 2^32^–1 (0x80000000
|
|
to 0xFFFFFFFF) are used _only_ for hardened derivation. Therefore, if
|
|
the index number is less than 2^31^, the child is normal, whereas if the
|
|
index number is equal or above 2^31^, the child is hardened.
|
|
|
|
To make the index number easier to read and display, the index number
|
|
for hardened children is displayed starting from zero, but with a prime
|
|
symbol. The first normal child key is therefore displayed as 0, whereas
|
|
the first hardened child (index 0x80000000) is displayed as 0++'++.
|
|
In sequence then, the second hardened key would have index 0x80000001
|
|
and would be displayed as 1++'++, and so on. When you see an HD
|
|
wallet index i++'++, that means 2^31^+i.
|
|
|
|
===== HD wallet key identifier (path)
|
|
|
|
((("hierarchical deterministic (HD) wallets")))Keys in an HD wallet are
|
|
identified using a "path" naming convention, with each level of the tree
|
|
separated by a slash (/) character (see <<table_4-8>>). Private keys
|
|
derived from the master private key start with "m." Public keys derived
|
|
from the master public key start with "M." Therefore, the first child
|
|
private key of the master private key is m/0. The first child public key
|
|
is M/0. The second grandchild of the first child is m/0/1, and so on.
|
|
|
|
The "ancestry" of a key is read from right to left, until you reach the
|
|
master key from which it was derived. For example, identifier m/x/y/z
|
|
describes the key that is the z-th child of key m/x/y, which is the y-th
|
|
child of key m/x, which is the x-th child of m.
|
|
|
|
[[table_4-8]]
|
|
.HD wallet path examples
|
|
[options="header"]
|
|
|=======
|
|
|HD path | Key described
|
|
| m/0 | The first (0) child private key from the master private key (m)
|
|
| m/0/0 | The first grandchild private key from the first child (m/0)
|
|
| m/0'/0 | The first normal grandchild from the first _hardened_ child (m/0')
|
|
| m/1/0 | The first grandchild private key from the second child (m/1)
|
|
| M/23/17/0/0 | The first great-great-grandchild public key from the first great-grandchild from the 18th grandchild from the 24th child
|
|
|=======
|
|
|
|
===== Navigating the HD wallet tree structure
|
|
|
|
The HD wallet tree structure offers tremendous flexibility. Each parent
|
|
extended key can have 4 billion children: 2 billion normal children and
|
|
2 billion hardened children. Each of those children can have another 4
|
|
billion children, and so on. The tree can be as deep as you want, with
|
|
an infinite number of generations. With all that flexibility, however,
|
|
it becomes quite difficult to navigate this infinite tree. It is
|
|
especially difficult to transfer HD wallets between implementations,
|
|
because the possibilities for internal organization into branches and
|
|
subbranches are endless.
|
|
|
|
Two BIPs offer a solution to this complexity by creating some proposed
|
|
standards for the structure of HD wallet trees. BIP43 proposes the use
|
|
of the first hardened child index as a special identifier that signifies
|
|
the "purpose" of the tree structure. Based on BIP43, an HD wallet
|
|
should use only one level-1 branch of the tree, with the index number
|
|
identifying the structure and namespace of the rest of the tree by
|
|
defining its purpose. For example, an HD wallet using only branch
|
|
m/i++'++/ is intended to signify a specific purpose and that
|
|
purpose is identified by index number "i."
|
|
|
|
Extending that specification, BIP44 proposes a multiaccount structure
|
|
as "purpose" number +44'+ under BIP43. All HD wallets following the
|
|
BIP44 structure are identified by the fact that they only used one
|
|
branch of the tree: m/44'/.
|
|
|
|
BIP44 specifies the structure as consisting of five predefined tree levels:
|
|
|
|
-----
|
|
m / purpose' / coin_type' / account' / change / address_index
|
|
-----
|
|
|
|
The first-level "purpose" is always set to +44'+. The second-level
|
|
"coin_type" specifies the type of cryptocurrency coin, allowing for
|
|
multicurrency HD wallets where each currency has its own subtree under
|
|
the second level. There are three currencies defined for now: Bitcoin is
|
|
m/44'/0', Bitcoin Testnet is m/44++'++/1++'++, and Litecoin is
|
|
m/44++'++/2++'++.
|
|
|
|
The third level of the tree is "account," which allows users to
|
|
subdivide their wallets into separate logical subaccounts, for
|
|
accounting or organizational purposes. For example, an HD wallet might
|
|
contain two bitcoin "accounts": m/44++'++/0++'++/0++'++
|
|
and m/44++'++/0++'++/1++'++. Each account is the root of
|
|
its own subtree.
|
|
|
|
((("keys and addresses", see="also public and private keys")))On the
|
|
fourth level, "change," an HD wallet has two subtrees, one for creating
|
|
receiving addresses and one for creating change addresses. Note that
|
|
whereas the previous levels used hardened derivation, this level uses
|
|
normal derivation. This is to allow this level of the tree to export
|
|
extended public keys for use in a nonsecured environment. Usable
|
|
addresses are derived by the HD wallet as children of the fourth level,
|
|
making the fifth level of the tree the "address_index." For example, the
|
|
third receiving address for bitcoin payments in the primary account
|
|
would be M/44++'++/0++'++/0++'++/0/2. <<table_4-9>> shows
|
|
a few more examples.
|
|
|
|
[[table_4-9]]
|
|
.BIP44 HD wallet structure examples
|
|
[options="header"]
|
|
|=======
|
|
|HD path | Key described
|
|
| M/44++'++/0++'++/0++'++/0/2 | The third receiving public key for the primary bitcoin account
|
|
| M/44++'++/0++'++/3++'++/1/14 | The fifteenth change-address public key for the fourth bitcoin account
|
|
| m/44++'++/2++'++/0++'++/0/1 | The second private key in the Litecoin main account, for signing transactions
|
|
|=======
|